Abstract
Phosphorylation on a serine or threonine residue preceding proline (Ser/Thr-Pro) is a key regulatory mechanism, and the conformation of certain phosphorylated Ser/Thr-Pro bonds is regulated specifically by the prolyl isomerase Pin1. Whereas the inhibition of Pin1 induces apoptosis, Pin1 is strikingly overexpressed in a subset of human tumours. Here we show that Pin1 regulates β-catenin turnover and subcellular localization by interfering with its interaction with adenomatous polyposis coli protein (APC). A differential-display screen reveals that Pin1 increases the transcription of several β-catenin target genes, including those encoding cyclin D1 and c-Myc. Manipulation of Pin1 levels affects the stability of β-catenin in vitro. Furthermore, β-catenin levels are decreased in Pin1-deficient mice but are increased and correlated with Pin1 overexpression in human breast cancer. Pin1 directly binds a phosphorylated Ser-Pro motif next to the APC-binding site in β-catenin, inhibits its interaction with APC and increases its translocation into the nucleus. Thus, Pin1 is a novel regulator of β-catenin signalling and its overexpression might contribute to the upregulation of β-catenin in tumours such as breast cancer, in which APC or β-catenin mutations are not common.
This is a preview of subscription content, access via your institution
Access options
Subscribe to this journal
Receive 12 print issues and online access
$209.00 per year
only $17.42 per issue
Buy this article
- Purchase on Springer Link
- Instant access to full article PDF
Prices may be subject to local taxes which are calculated during checkout








Similar content being viewed by others
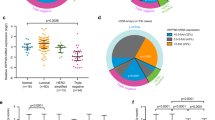
References
Kinzler, K. W. & Vogelstein, B. Lessons from hereditary colorectal cancer. Cell 87, 159–170 (1996).
Polakis, P. Wnt signaling and cancer. Genes Dev. 14, 1837–1851 (2000).
Morin, P. J. β-Catenin signaling and cancer. BioEssays 21, 1021–1030 (1999).
Kinzler, K. W. et al. Identification of FAP locus genes from chromosome 5q21. Science 253, 661–665 (1991).
Su, L. K., Vogelstein, B. & Kinzler, K. W. Association of the APC tumor suppressor protein with catenins. Science 262, 1734–1737 (1993).
Rubinfeld, B. et al. Association of the APC gene product with β-catenin. Science 262, 1731–1734 (1993).
Korinek, V. et al. Constitutive transcriptional activation by a β-catenin–Tcf complex in APC−/− colon carcinoma. Science 275, 1784–1787 (1997).
Morin, P. J. et al. Activation of β-catenin-Tcf signaling in colon cancer by mutations in β-catenin or APC. Science 275, 1787–1790 (1997).
Rubinfeld, B., Albert, I., Porfiri, E., Munemitsu, S. & Polakis, P. Loss of β-catenin regulation by the APC tumor suppressor protein correlates with loss of structure due to common somatic mutations of the gene. Cancer Res. 57, 4624–4630 (1997).
Munemitsu, S., Albert, I., Souza, B., Rubinfeld, B. & Polakis, P. Regulation of intracellular β-catenin levels by the adenomatous polyposis coli (APC) tumor-suppressor protein. Proc. Natl Acad. Sci. USA 92, 3046–3050 (1995).
Behrens, J. et al. Functional interaction of β-catenin with the transcription factor LEF-1. Nature 382, 638–642 (1996).
Molenaar, M. et al. XTcf-3 transcription factor mediates β-catenin-induced axis formation in Xenopus embryos. Cell 86, 391–399 (1996).
He, T. C. et al. Identification of c-MYC as a target of the APC pathway. Science 281, 1509–1512 (1998).
He, T. C., Chan, T. A., Vogelstein, B. & Kinzler, K. W. PPARδ is an APC-regulated target of nonsteroidal anti-inflammatory drugs. Cell 99, 335–345 (1999).
Tetsu, O. & McCormick, F. β-Catenin regulates expression of cyclin D1 in colon carcinoma cells. Nature 398, 422–426 (1999).
Gradl, D., Kuhl, M. & Wedlich, D. The Wnt/Wg signal transducer β-catenin controls fibronectin expression. Mol. Cell. Biol. 19, 5576–5587 (1999).
Rubinfeld, B. et al. Binding of GSK3β to the APC–β-catenin complex and regulation of complex assembly. Science 272, 1023–1026 (1996).
Kitagawa, M. et al. An F-box protein, FWD1, mediates ubiquitin-dependent proteolysis of β-catenin. EMBO J. 18, 2401–2410 (1999).
He, X. et al. Glycogen synthase kinase-3 and dorsoventral patterning in Xenopus embryos. Nature 374, 617–622 (1995).
Henderson, B. R. Nuclear–cytoplasmic shuttling of APC regulates β-catenin subcellular localization and turnover. Nature Cell Biol. 2, 653–660 (2000).
Neufeld, K. L., Zhang, F., Cullen, B. R. & White, R. L. APC-mediated downregulation of β-catenin activity involves nuclear sequestration and nuclear export. EMBO Rep. 1, 519–523 (2000).
Rosin-Arbesfeld, R., Townsley, F. & Bienz, M. The APC tumour suppressor has a nuclear export function. Nature 406, 1009–1012 (2000).
Jonsson, M., Borg, A., Nilbert, M. & Andersson, T. Involvement of adenomatous polyposis coli (APC)/β-catenin signalling in human breast cancer. Eur. J. Cancer 36, 242–248 (2000).
Lin, S. Y. et al. β-Catenin, a novel prognostic marker for breast cancer: its roles in cyclin D1 expression and cancer progression. Proc. Natl Acad. Sci. USA 97, 4262–4266 (2000).
Schlosshauer, P. W. et al. APC truncation and increased β-catenin levels in a human breast cancer cell line. Carcinogenesis 21, 1453–1456 (2000).
Roose, J. et al. Synergy between tumor suppressor APC and the β-catenin-Tcf4 target Tcf1. Science 285, 1923–1926 (1999).
Wulf, G. M. et al. Pin1 is overexpressed in breast cancer and potentiates the transcriptional activity of phosphorylated c-Jun towards the cyclin D1 gene. EMBO J. 20, 3459–3472 (2001).
Lu, K. P., Hanes, S. D. & Hunter, T. A human peptidyl-prolyl isomerase essential for regulation of mitosis. Nature 380, 544–547 (1996).
Ranganathan, R., Lu, K. P., Hunter, T. & Noel, J. P. Structural and functional analysis of the mitotic peptidyl-prolyl isomerase Pin1 suggests that substrate recognition is phosphorylation dependent. Cell 89, 875–886 (1997).
Yaffe, M. B. et al. Sequence-specific and phosphorylation-dependent proline isomerization: a potential mitotic regulatory mechanism. Science 278, 1957–1960 (1997).
Zhou, X. Z. et al. Pin1-dependent prolyl isomerization regulates dephosphorylation of Cdc25C and tau proteins. Mol. Cell 6, 873–883 (2000).
Shen, M., Stukenberg, P. T., Kirschner, M. W. & Lu, K. P. The essential mitotic peptidyl-prolyl isomerase Pin1 binds and regulates mitosis-specific phosphoproteins. Genes Dev. 12, 706–720 (1998).
Lu, P. J., Zhou, X. Z., Shen, M. & Lu, K. P. A function of WW domains as phosphoserine- or phosphothreonine-binding modules. Science 283, 1325–1328 (1999).
Lu, P. J., Wulf, G., Zhou, X. Z., Davies, P. & Lu, K. P. The prolyl isomerase Pin1 restores the function of Alzheimer-associated phosphorylated tau protein. Nature 399, 784–788 (1999).
Zhou, X. Z., Lu, P. J., Wulf, G. & Lu, K. P. Phosphorylation-dependent prolyl isomerization: a novel signaling regulatory mechanism. Cell. Mol. Life Sci. 56, 788–806 (1999).
Rippmann, J. F. et al. Phosphorylation-dependent proline isomerization catalyzed by Pin1 is essential for tumor cell survival and entry into mitosis. Cell Growth Differ. 11, 409–416 (2000).
Kondoh, N. et al. A method to isolate differentially expressed genes by displaying specific inner portion of cDNA fragments. Anal. Biochem. 269, 427–430 (1999).
Ryo, A. et al. Identification and characterization of differentially expressed mRNAs in HIV type 1-infected human T cells. AIDS Res. Hum. Retrovir. 16, 995–1005 (2000).
Fujimori, F., Takahashi, K., Uchida, C. & Uchida, T. Mice lacking Pin1 develop normally, but are defective in entering cell cycle from G(0) arrest. Biochem. Biophys. Res. Commun. 265, 658–663 (1999).
Murray, A. W. Cell cycle extracts. Methods Cell Biol. 36, 581–605 (1991).
Graham, T. A. et al. Crystal structure of a β-catenin/Tcf complex. Cell 103, 885–896 (2000).
von Kries, J. P. et al. Hot spots in β-catenin for interactions with LEF-1, conductin and APC. Nature Struct. Biol. 7, 800–807 (2000).
Neufeld, K. L. et al. Adenomatous polyposis coli protein contains two nuclear export signals and shuttles between the nucleus and cytoplasm. Proc. Natl Acad. Sci. USA 97, 12085–12090 (2000).
Espada, J. et al. H-Ras activation promotes cytoplasmic accumulation and phosphoinositide 3-OH kinase association of β-catenin in epidermal keratinocytes. J. Cell Biol. 146, 967–980 (1999).
Playford, M. P., Bicknell, D., Bodmer, W. F. & Macaulay, V. M. Insulin-like growth factor 1 regulates the location, stability, and transcriptional activity of β-catenin. Proc. Natl Acad. Sci. USA 97, 12103–12108 (2000).
Giannini, A. L., Vivanco, M. M. & Kypta, R. M. Analysis of β-catenin aggregation and localization using GFP fusion proteins: nuclear import of α-catenin by the β-catenin/Tcf complex. Exp. Cell Res. 255, 207–220 (2000).
Kim, K., Pang, K. M., Evans, M. & Hay, E. D. Overexpression of β-catenin induces apoptosis independent of its transactivation function with LEF-1 or the involvement of major G1 cell cycle regulators. Mol. Biol. Cell 11, 3509–3523 (2000).
Simcha, I. et al. Differential nuclear translocation and transactivation potential of β-catenin and plakoglobin. J. Cell Biol. 141, 1433–1448 (1998).
Lu, K. P. & Hunter, T. Evidence for a NIMA-like mitotic pathway in vertebrate cells. Cell 81, 413–424 (1995).
Nakamura, M., Zhou, X. Z. & Lu, K. P. Critical role for the EB1 and APC interaction in the regulation of microtubule polymerization. Curr. Biol. 11, 1062–1067 (2001).
Acknowledgements
We thank M. Yamamoto for providing reagents for the initial differential display screen; X. He for discussions; T. Hunter for Pin1 knockout mice; I. Kosugi for technical instructions; B. Vogelstein, X. He, R. Pestell and S. Hatakeyama for reagents; and X. Zhou, O. Kops and P. J. Lu in the Lu laboratory for their important contributions. A.R., G.W., Y.-C.L. and N.M. are fellows of the Japan Society for the Promotion of Science, the DOD Breast Cancer Research Program, the National Sciences and Engineering Research Council of Canada and the Human Frontier Research Program, respectively. K.P.L. is a Pew Scholar and a Leukemia and Lymphoma Society Scholar. This study was supported the NIH grants to K.P.L.
Author information
Authors and Affiliations
Corresponding author
Supplementary information
Figure S1
β-Catenin structure and its binding sites for APC and Pin1. (PDF 118 kb)
Rights and permissions
About this article
Cite this article
Ryo, A., Nakamura, M., Wulf, G. et al. Pin1 regulates turnover and subcellular localization of β-catenin by inhibiting its interaction with APC. Nat Cell Biol 3, 793–801 (2001). https://doi.org/10.1038/ncb0901-793
Received:
Revised:
Accepted:
Published:
Issue Date:
DOI: https://doi.org/10.1038/ncb0901-793
This article is cited by
-
PIN1 and CDK1 cooperatively govern pVHL stability and suppressive functions
Cell Death & Differentiation (2023)
-
Hyperglycemia induces gastric carcinoma proliferation and migration via the Pin1/BRD4 pathway
Cell Death Discovery (2022)
-
Ligand-specific conformational change drives interdomain allostery in Pin1
Nature Communications (2022)
-
Impact of PIN1 Inhibition on Tumor Progression and Chemotherapy Sensitivity in Colorectal Cancer
Journal of Gastrointestinal Cancer (2022)
-
Screening of the HBx transactivation domain interacting proteins and the function of interactor Pin1 in HBV replication
Scientific Reports (2021)